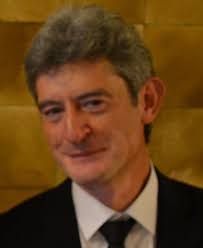
Decarbonization of the existing heating and cooling networks by the introduction of ground source heat pumps
Heat pumps vs. fossil fuels
Worldwide some 4.5 million people die prematurely every year due to air pollution generated by burning fossil fuels and the increased levels of particulate matter PM2.5, while the overall cost is estimated as 3.3% of world GDP[1]. A recent study[2] also relates the mortality of Covid-19 with the long-term exposure to air pollution and PM2.5. This means that a small increase in PM2.5 exposure (air pollution) has 20 times more lethal impact in Covid-19 death rate. Therefore, a decarbonization of our existing energy networks, based primarily on fossil fuels generation, is a necessity for sustainable and healthy future.
One of the most effective ways to reduce air pollution in cities and to cut carbon emissions is by integrating ground source heat pumps (GSHP) within the existing energy networks, nowadays primarily based on fossil fuels in global scale. In Finland, there is very few municipal/district-scale projects and research covering this topic. Nowadays, heat pumps’ deployment in Finland is mostly driven by small air-/ground-source configurations in dwellings. Unfortunately, there are still only few large-scale utility realizations taking advantage of GSHP in combination with underground thermal energy storage (UTES).
Energy storage and the power of GSHP-UTES tandem
Annual mismatch between buildings´ energy demand and renewable generation is a well-known issue in energy management. Normally heat is supplied in winter whereas in summer excess heat (cooling) is typically wasted, resulting in inefficient overall operation. Thus, seasonal energy storage is a necessity for balancing supply and demand as well as for increasing the overall system flexibility. The ground beneath us is a perfect supplement for GSHPs since below 10 meters from the surface it maintains a roughly constant temperature, slightly higher than the average annual temperature of the place. In cities, the undisturbed ground temperature is even higher (some 4-7 oC higher than the average), due to the so called subsurface urban heat island effect (SUHI). In Nordic countries, given that heating operation dominates, higher ground-source temperature is an additional advantage in cities, also increasing the performance of heat pumps (COP).
The most utilized possibilities for underground thermal energy storage (UTES) are aquifer thermal energy storage (ATES) as an open-loop option, and borehole thermal energy storage (BTES) used in closed-loop. Wherever the hydrogeological conditions are favorable, ATES utilization is an attractive technological possibility. It is suitable for large buildings and utilities as well as capable to enable important thermal storage capacities.
Globally, some 3000 ATES systems are operated worldwide[3]. The Netherlands with 85% of all ATES realizations, followed by Sweden, Denmark and Belgium, are the undisputed frontrunners. Nowadays, overall ATES suitability[4] in the Nordic countries (Finland, Sweden and Norway) is estimated as 3-5 (out of 10). Moreover, ATES suitability in Finland will increase to 5-7 in the forthcoming decades due to climate change. Unfortunately, in Finland there is still not a relevant number of ATES implementations.
Current status of global ATES application, map extracted from the research of Fleuchaus et al. (2018)
GSHP-ATES integration for district heating and cooling
Two recent publications analyze the integration of ground source heat pumps (GSHP) within the existing district heating and cooling networks by introducing two case studies in Finland; Pukkila[5] and Kupittaa[6] (Turku). Both journal articles emphasize the importance of GSHP in tandem with UTES for efficient and sustainable decarbonization of the existing district energy networks. They also deepen on how GSHP-ATES integration can be done in practice and propose a hands-on methodology for technoeconomic evaluation, modeling and analysis of the long-term impact. The main results can be summarized as follows:
- methodology for the utilization of available Finnish open data sources related to hydrogeological and geographic conditions
- mathematical and groundwater modeling of GSHP-ATES operation
- development of different case studies analyzed in terms of efficiency and technoeconomic feasibility
- simulation and analysis of the long-term environmental impact of GSHP-ATES operation
The main conclusion is that dispatching heating and cooling loads in a single operation with annually balanced ATES management in terms of energy and pumping flows results in a low long-term environmental impact, being also economically feasible especially in a district level (energy production cost below 30 €/MWh in the case of Kupittaa).
Overall, GSHP-ATES systems prove to be a sustainable and efficient alternative to traditional thermal energy generation based primarily on fossil fuels. This is due to their ability to recycle heating and cooling loads using the subsurface as practically unlimited thermal storage within integrated district energy networks, especially in urban areas.
Conclusions
Humanity is facing difficult times: the foundations of our social and economic systems are dangerously challenged by a single virus. The way we respond and overcome this crisis will shape our future society during the forthcoming decades. In this context, our current energy systems, primarily based on the utilization of fossil fuels, are responsible for air pollution and premature deaths as well as jeopardize the future of our planet. The reduction of carbon emissions and overall pollution is a necessity – especially in cities – and ground source heat pumps (GSHP) in tandem with underground thermal energy storage (UTES) can play a crucial role for efficient and sustainable decarbonization of our district energy networks.
The future transition to low temperature district heating networks by the introduction of GSHP-ATES can eventually benefit from the proposed modeling methodology, specifically developed for Finnish conditions through two different case studies. This is due to its capability to find a trade-off between the system’s technical constraints, overall technoeconomic feasibility and the long-term environmental impact.
Oleg Todorov, M.Sc.(Tech) and M.Sc.(Arch)
Aalto University, Department of Mechanical Engineering
[1] Greenpeace (2020): Toxic air: The price of fossil fuels, February 2020: https://www.greenpeace.org/usa/wp-content/uploads/2020/02/The-Price-of-Fossil-Fuels-media-briefing.pdf
[2] Wu, X. et al. (2020). Exposure to air pollution and COVID-19 mortality in the United States. MedRxiv. https://doi.org/10.1101/2020.04.05.20054502
[3] Fleuchaus, et al. (2018). Worldwide application of aquifer thermal energy storage – A review. Renewable and Sustainable Energy Reviews, pp. 861-876. https://doi.org/10.1016/j.rser.2018.06.057
[4] Bloemendal et al. (2015). Combining climatic and geo-hydrological preconditions as a method to determine world potential for aquifer thermal energy storage. The Science of the total environment, 538, pp. 621-633. https://doi.org/10.1016/j.scitotenv.2015.07.084
[5] Todorov et al. (2020) A method and analysis of aquifer thermal energy storage (ATES) system for district heating and cooling: A case study in Finland”, Sustainable Cities and Society Volume 53, February 2020, 101977; https://doi.org/10.1016/j.scs.2019.101977
[6] Todorov et al. (2020) Aquifer thermal energy storage (ATES) for district heating and cooling: A novel modeling approach applied in a case study of a Finnish urban district”. Energies 2020, 13(10), 2478; Included in a Special Issue “Municipal Energy System Planning: New Approaches, Applications and Future Research Needs”, Energies, May 2020; https://doi.org/10.3390/en13102478